Introduction
There are many geographic, social, economic and
technological challenges that K-College science
educators and students regularly face (Burns, 1995).
Two of the most pressing ones are the continuing
expansion of the Intergenerational Information
Technology (IIT) divide and the social demand for
broad, comprehensive and balanced multidisciplinary
education.
Since 2000, we have witnessed the explosion of the
Internet, various web-applications and information
technologies for content generation and delivery.
Certain groups of the population help develop and
embrace these new ITs, others just try to keep
abreast with them and still others are barely
hanging in, if not completely giving up on the new
IT developments (Inoue, 2006). There are extremely
strong age effects in how the population perceives
and responds to these technological demands, changes
and implications (Finegold, Mohrman, & Spreitzer,
2002). Typically, students and learners are more
inclined to be engaged in development, testing and
adoption of new ITs (Maag, 2006; Yasar, Little,
Tuzun, Rajasethupathy, Maliekal, & Tahar, 2006).
Despite their frequent early exposure to new IT and
blended educational developments, teachers and
instructors seem to be much more conservative, less
willing to explore, validate and/or adopt new IT
instruments in their curricula (Koukel, 2006;
Vannatta & Fordham, 2004), e.g.,
www.genyes.com,
www.eschoolnews.com. This leads to an undesired
gap – the Intergenerational IT divide – between the
providers (instructors) and consumers (learners) of
educational and training curricula. This effect is
secondary to the normative gap representing the
natural gradient in the knowledge of the two parties
engaged in science education. The knowledge-gap is
an important underlying cause for our educational
endeavors – to bring up the next generation of
citizens, well prepared to deal with future
scientific, health, political, environmental or
social challenges. The IIT divide is a byproduct of
the fast-paced evolution of technology, which will
likely widen and have detrimental effects on the
ways we teach, the efficacy and apprehension of our
educational endeavors.
The second considerable challenge in modern science
education is the training of complete and broadly
educated workforce. On the short-term, it may be
economical, resourceful or appealing to train
students extremely well in one narrow scientific
discipline or subject. We may accomplish much
specific progress, with measurable outcomes, by
allocating significant resources in development of
an individual-field expertise. However, the
long-term results of such approach may be quite the
opposite of expectations. Now-a-days, most graduate
and professional schools, as well as much of the job
opportunities, seek to recruit individuals that have
widespread interests, training and capabilities. For
example, application review panels at top medical
schools currently give preferences to applicants
that have a round background and affinity to social,
geo-political, scientific and cultural facts and
affairs; more so than to applicants that may be
exceptionally strong in only one discipline or have
narrowly defined curiosity. For example,
UCSD School of Medicine admission guidelines state A
broad base of knowledge is advantageous in preparing
[to be] a physician;
Harvard Medical School is looking for people with
broad interests and talents;
University of Pennsylvania School of Medicine
advises applicants to consider and think broadly of
[their prior] academic and extra-curricular
experiences
This comes from the realization that a modern clinician, scientist,
engineer, economist or politician needs to be able
to reason, integrate knowledge and make decisions in
out-of-the-box situations, where no single
discipline or methodology is likely to solve a
problem completely. In addition, abilities to
effectively navigate and bridge the gaps between
different science disciplines, social orders and
behavioral psychologies will likely improve the
prospects for our future workforce, leaders and
scientists (Munshi, 2006). Lastly, the transfer and
blending of data, research challenges and techniques
between diverse areas of science is critical in
motivating majority of students, demonstrating
cross-disciplinary methodological concepts and
synergies as well as for engaging students in
research projects.
If technology enhanced multidisciplinary education
improves student motivation and learning retention,
what are some specific instances of IT instruments
that may be employed by instructors? Modern
information technology-driven educational tools are
much more than simply collections of static lecture
notes and homework assignments posted on one
course-specific Internet site. Over the past five
years, a number of technologies have emerged that
provide dynamic, linked and interactive learning
content with heterogeneous points-of-access to
educational materials (Dinov, Sanchez, & Christou,
2008) These new IT resources include common
web-places for course materials (e.g., Blackboard,
Moodle), complete online courses (e.g.,
http://www.uclaextension.edu/), Wikis (e.g.,
http://wiki.stat.ucla.edu/socr), interactive
video streams (e.g.,
http://duber.com/LetsTalk,
http://blog.click.tv/,
www.ivtweb.com/),
audio-visual classrooms, real-time educational blogs
(e.g.,
http://www.pbs.org/teachersource/learning.now),
(Brescia & Miller, 2006), web-based resources for
blended instruction (e.g.,
http://en.wikibooks.org/wiki/Blended_Learning_in_K-12),
virtual office hours with instructors (e.g.,
http://voh.chem.ucla.edu/), collaborative
learning environments (e.g.,
http://sakaiproject.org/), test-banks and
exam-building tools (e.g.,
http://sourceforge.net/projects/tcexam/) and
resources for monitoring and assessment of learning.
Challenges and Actions
No one can accurately predict the technological
advancements or the prospective pedagogical
challenges that educators will have to deal with in
the next 10-30 years. This is because of the
expected exponential increase in computational power
and significant geopolitical, social and
environmental challenges we are likely to face in
this period. Yet, it is reasonable to assume
that the technological progress we are likely to see
will exceed an order of magnitude the advances
witnessed in the past 20 years (Adomavicius,
Bockstedt, Gupta, & Kauffman, 2006; Munakata, 2007;
Pareek, 2006; Roberts, 2000). Such rapid increases
in knowledge build up, data collection,
communication and information infrastructure, for a
relatively short time-span, will strain the delicate
but functional balance we had reached in the 20th
century in educating a broadly intelligent and
skillful workforce. Two specific high-impact science
educational challenges are likely to exacerbate over
the next couple of decades – the Intergenerational
IT divide between students and educators; and the
dilemma of training broadly educated citizens at
higher cost vs. the alternative of lower-cost
discipline-specific education.
Intergenerational IT Divide
The accelerated development and utilization of the
Internet, web-applications and new information
technologies in all areas of social life has also
impacted the field of science education. There is an
interesting interplay between technology developers
(visionaries and engineers) and the general public.
New technologies may be designed by developers to
solve general problems or may be introduced to solve
some specific user needs. In either situation, age
effects may sometimes have desirable taming
implications or unintended forestalling
repercussions (Daveri & Maliranta, 2007; Willis &
Tranter, 2006). Younger learners are more willing to
get involved in design, testing and broad
distribution of new ITs (Bongalos, Bulaon, Celedonio,
de Guzman, & Ogarte, 2006). There is evidence
suggesting a correlation between the use of IT in
the classroom and the level of student involvement (Cutrim,
Rudge, Kits, Mitchell, & Nogueira, 2006). On the
other hand, instructors appear to be more attracted
to the established/accepted pedagogical techniques
than to explore newly introduced IT instruments (Cartelli,
2006; Guillot & Pryor, 2007; Moore, 2006). Certainly
the majority of new technologies may eventually be
discarded, proven to be inefficient or be quickly
replaced by even newer advancements. However, some,
like the collaborative Wiki environments, are bound
to make an impact on how we train the next
generation of scientists and engineers. This IIT gap
between the educators and students is the result of
differences in perceptions of the goals of
scientific training, socio-economic factors, as well
as quick turn around of technological breakthroughs
(Conole, 2008). Left unchecked, the IIT gap will
likely expand and i on the immediate efficacy
and long-term apprehension of our current
educational activities.
There are several possible strategies to slow and perhaps reverse the IIT
divide and ensure that our educational efforts are
balanced and effective. Some of these are discussed
below.
Continuing Instructional Technology Training
One of the most powerful ways to impact the
progression of the IIT divide is to involve science
educators in continuing technology education
training. This may be done under discipline-specific
or general societal/organizational umbrellas. Most
instructors and teachers already participate in
various semiannual or quarterly continuing education
events within their discipline. Few are deeply
involved in regular technology-based refresher
courses. For example, fewer that 25% of the UCLA
statistics faculty attend a continuing education
course each year. The experience of this author, as
a recipient and deliverer of science and technology
education to teachers and educators, confirms that
many instructors are not familiar with the basic
modern means of Internet communication (including,
blogging, collaborative wiki environments, database
and applet interfaces, web-services, data resources,
etc.) A sustained effort is needed on the part of
educators, science administrators, policy makers and
the general public to facilitate and enable
continuing science and technology education for
instructors at all levels.
Engagement of Instructors in Science and
Technology Research Projects
Instructors from elementary school to college should
be involved in science and technology research
projects pertinent to their level and curricula. By
judging at variety of state and local science fairs,
this author has been very impressed by the
motivation, elaborate thinking and complexity of
projects developed by K-College students who were
supervised by instructors engaged in
multi-disciplinary science and technology research.
For instance, the annual California Science Fair
serves as a forum for showcasing student research
projects directed by K-12 teachers and college
instructors, a venue for exchange of ideas and as an
informal student project evaluation mechanism. The
amazing variety and work quality of student projects
presented there are evidence of the effects of
engaging instructors in research projects (e.g.,
http://www.usc.edu/CSSF/History/2006/Alpha.html).
There are various ways to learn and contribute to
new or ongoing research projects conducted by higher
education institutions, professional organizations,
private and federally funded initiatives (e.g.,
www.usc.edu/CSSF,
http://NationalGeographic.com/genographic,
ed.fnal.gov/trc_new/projects/web_resources.shtml,
etc.)
Institutional Commitment in IT and Blended
Instruction
The interest, available infrastructure and resources
provided by home institutions are essential for the
engagement of teachers and instructors in
contemporary science and technology education. Many
institutions (e.g., schools, colleges, universities,
institutes and centers) provide computational
resources, audio-visual and Internet-digital
infrastructure, seed grants, human resources and
other forms of support to entice their instructors
in technology learning and creative utilization of
IT in the classroom (e.g.,
www.oid.UCLA.edu/AVS). Institutional commitment
could be a significant barrier or a considerable
asset in developing an IT blended curriculum.
Funding Agency Engagement in IT and Blended
Education
There are a multitude of agencies that provide
funding support for a wide spectrum of innovative
educational endeavors (e.g.,
www.ED.gov,
www.GrantsAlert.com,
www.NSF.gov,
www.spencer.org,
www.kidsinneed.net, etc.) Federal, state and
private funding initiatives aim at stimulating
creative thinking and exploration of novel
strategies for IT and blended education. Even though
these are merit based and in some instances very
competitive, few good proposals are turned down. For
example, in 2006-2007, the National Science
Foundation received 44,000 grants, 11,000 of which
were funded (of course, not all were education
related). When submitting a grant proposal, the
critical components are to find a specific call for
application that jibes well with the proposed idea,
format the proposal according to the specific call
and grant writing guidelines, and ensure that the
proposal addresses a real (educational) challenge
that is not already solved. Such extramural funding
does enable instructors to buy out time for
attending continuing education events, develop
blended curricula, establish new collaborations,
collect data and design research projects. All of
these will have a positive effect on reducing the
IIT gap.
Integration of Available Digital Resources in the
Classroom
In the past several years, a large number of digital
resource libraries have been cataloging, curating,
evaluating and integrating most of the valuable IT
resources for enhancing science education using
interactive aids, electronic media and instructional
materials. A list of some of these resource
libraries is available online at
http://SOCR.ucla.edu/htmls/SOCR_Recognitions.html.
These resources provide datasets, methodological and
conceptual learning materials, tools for data
analysis and exploration, hands-on activities,
demonstrations, tutorials and refreshers for all
disciplines, topics and levels. Instructors should
dedicate the time to find, test, compare and select
appropriate resources for their curricula. With
respect to web utilization, students are much ahead
of most instructors and they can easily discover
Internet resources on their own (Maag, 2006; Yasar
et al., 2006). The problem with the latter is that
students may use inappropriately such resources
(intentionally or unintentionally) without
instructors even realizing it. For example, many
instructors (including this author) are sometimes
unaware that on the Internet, problem solutions,
virtual tutors and blogs contain solutions to many
homework assignments, which diminishes the intended
value of personally completing such assignments. The
reduction of the IIT gap will taper these
possibilities and increase the value of personal
learning efforts.
Broad vs. Narrow Spectrum Educational Training
There is a good agreement that all learners need to
be generally trained first (Hernon & Schwartz,
2006; Posch & Steiner, 2006). Science oriented
learners eventually should branch out and specialize
in a specific discipline that they further explore
and advance in. The main question is: when should
the regression into a specific scientific area occur
in the learner’s course of training? In the past,
this break point was probably in the first decade of
life. More recently, the point of specialization
occurred first in high-school (1960’s), then in
college (1980-1990’s). Now-a-days, science students
frequently carry multi-disciplinary research and
training in graduate and post-graduate school. This
ever increasing demand for multidisciplinary
training reflects the steady increase in human
life-span, the accumulation of general knowledge,
technological advancements and the escalation of the
complexity of problems addressed by researchers,
clinicians and policy-makers.
The debate over the discipline bound vs. cross-discipline training is
especially crucial in undergraduate and graduate
college education (Holt, 2007; Mitrany & Stokols,
2005). Frequently, the two extremes are pure
mathematics students (very discipline-oriented,
high-level of competency, low cost training) and
various humanitarian majors (broad discipline
learning requirements, including many watered-down
quantitative courses, somewhat higher training
costs, low-level knowledge spanning many subjects).
The multi-disciplinary and area-specific educational
trainings are complementary, not overlapping or
antagonistic. For example, brain mapping and
computational neuroscience challenges may serve as
driving biological projects for developing novel
methods for statistical analysis (Dinov, 2005). And
conversely, the introduction of new mathematical
models of shape may lead to unexpected biomedical
applications (Srivastava, 2005). An over commitment
to any one of the two educational directives will
ultimately have drastically negative effect on the
entire educational system. The reason is that
multi-disciplinary sciences are completely
contingent upon having robust and computationally
tractable theoretical properties and results needed
to establish the spokes of modern scientific
understanding of the physical world we live in.
Multidisciplinary research efforts allow us to build
bridges and establish affinities between such
(sometimes independent) spokes of scientific
endeavors. On the other hand, no single scientific
area can model, represent and explain the
complexities in any physical, biological, social,
political, medical or psychological system.
Solutions to all real-world problems demand
multi-disciplinary thinking and cross-scientific
approaches.
Some of the directions below may help us promote interdisciplinary
science and protect the homeostasis between
discipline-oriented and cross-discipline thrusts in
science education.
Develop New and Diverse Multidisciplinary
Educational Curricula
There is a real need to design new plans for
multidisciplinary instruction involving two or more
disciplines. The lack of available educational
materials that cross between different disciplines
and enable sharing of data, techniques and tools is
due to scarcity of expertise to develop such
instruments (Borgman, 2006). Few educators are well
versed in multiple scientific fields (Bender, 2005;
Freeman, 2005). Thus, collaborations and
interactions between different areas are sometimes
impeded by insurmountable linguistic, methodological
and physiological barriers (e.g., terminology,
specifications of data and methodological protocols,
a priori assumptions). The community of science
educators should strive to improve communication and
overhaul the efforts to develop educational
materials and transferable knowledge across multiple
disciplines. Examples of such efforts involving
mathematicians, engineers, biologists, statisticians
and physicists are developed by the
National
Internet-based Science Educational Resource.
Institutional Commitment to Multidisciplinary
Education
Little progress in designing, validating and
disseminating multidisciplinary educational
materials is practically possible before ensuring
firm institutional commitments and support to these
efforts. In addition to demanding scientific
expertise from varieties of fields,
multidisciplinary resource developments also require
logistical, financial, administrative and human
resource commitments that are only conceivable and
implementable by larger institutional,
organizational and legislative initiatives. A 2004
National Academy of Sciences report entitled
Facilitating Interdisciplinary Research (NAS, 2004)
identified barriers to interdisciplinary efforts
including limited resources, the academic reward
system, differences in disciplinary cultures, the
pursuit of national rankings (based on traditional
disciplinary categorizations), differences in
policies and procedures across departments, and
decentralized budget strategies that give advantages
to departments over interdisciplinary programs.
The University of California, Los Angles (UCLA) is one example of an
organization embracing crosscutting educational
programs that serve undergraduates and graduates.
UCLA includes 32 interdepartmental program majors,
nearly 500 courses are offered as cross-listed in
two or more departments, faculty members participate
in several departments through split appointments
(5.4%) or joint appointments (24.5%), and there are
over 80 campus-based multidisciplinary research
centers, characterized by long-term institutional
commitment and robust funding.
Introduce Efficient Multidisciplinary Resource
Interfaces
Even if we are successful in developing, testing and
distributing a large number of interdisciplinary
educational resources, a possible imperative to
their utilization by instructors as classroom aids
may be the efficient means of resource traversal,
querying and comparisons. Such interfaces to
multidisciplinary digital libraries should
facilitate the community involvement in updating,
ranking and management of the resource collectives.
NSDL,
MathDL, and
NISER provide examples of such
interfaces. This figure below illustrates an example
of the interactive and dynamic exploration of the
NISER resources. The issues of classification,
organization, traversal and management of
interdisciplinary materials and educational
resources will become pressing with the expansion of
the volume, complexities and affinities of these
resources. Efficient, robust and extensible
infrastructures for management, visualization and
(human and machine) interaction with these resources
will be vital for the success of the future
interdisciplinary educational efforts.
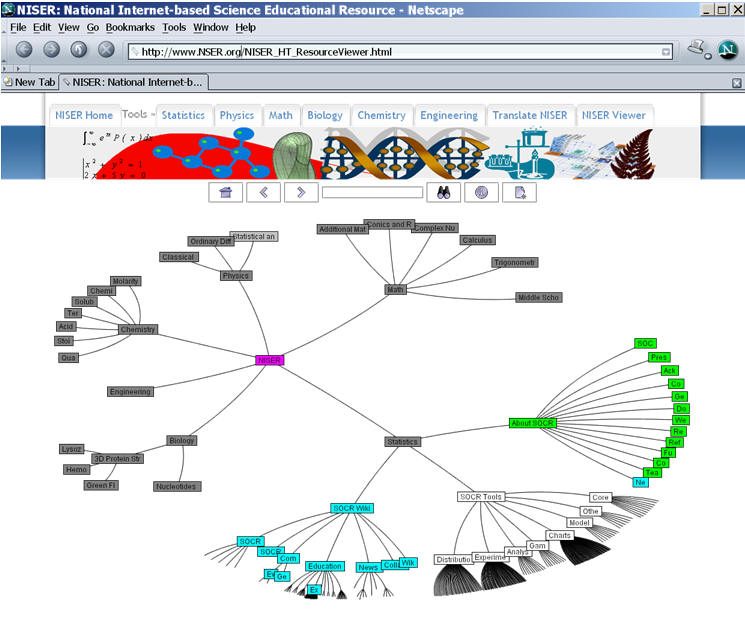
The NISER interactive graphical user interface
enabling traversal, discovery and dynamic
exploration of available multidisciplinary
educational resources and learning materials
(http://www.nser.org/NISER_HT_ResourceViewer.html).
Expectations of Student Participation in
Multidisciplinary Programs
There are several direct implications of engaging
students in multidisciplinary learning activities.
Interdisciplinary training exposes students to the
practical interactions of concepts and properties
they have learnt in other field-specific curricula.
This reinforces the meaning and applications of
these concepts to study natural processes or develop
models about our world. Multidisciplinary research
projects also tend to rapidly and sustainably
attract students’ attention and serve as a powerful
learning motivational tool (Joan, 2007). Learners
exposed to well-designed and interactive hands-on
multidisciplinary training acquire unique
perspectives in understanding and dealing with
complex problems (Shekar, 2007). Such training
provides the glue that will hold together and
perhaps stimulate the future scientific endeavors.
At the same time, imminent advancement in scientific
research and applications is contingent upon the
availability of sufficient high-level knowledge
expertise within each scientific field as well as
the abilities of researchers and scientists to look
across many areas, draw resources and make inference
about general multi-disciplinary challenges.
Mechanisms for Assessment of Instructional
effectiveness of Interdisciplinary Education
Quantitative evaluations of many educational
interventions, including the efficacy of
multidisciplinary training, are difficult because
treatment effects may be observed as dependent or
categorical measurements, they may be significantly
delayed in time, be extremely
non-parametric/non-linear or may be indirectly
manifested (Dinov et al., 2008). Therefore, a
mixture of quantitative and qualitative evidence
should be used to assess the effects of
interdisciplinary training, as well as the social
and economic impacts of the balance between
high-competency area-bound and cross-disciplinary
education. The table below summarizes some of the
important measures for assessment of the efficacy,
perception and promise of interdisciplinary
education to improve human life, health and general
knowledge.
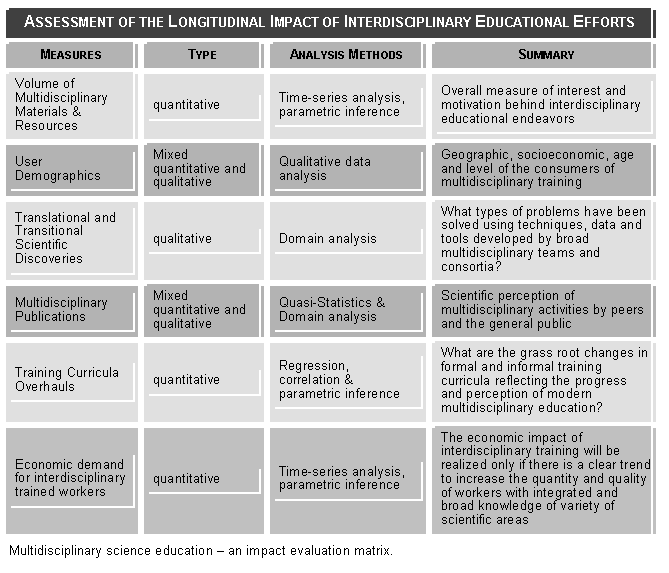
Discussion
There will be some educational challenges in the
next decade that we can foresee now and many that
will only become apparent in the future. For
instance, it is expected that computers and Internet
usage will vary by family socioeconomic status, race
and parent educational level (Norris, 2001). There
are several studies that have consistently
established this notion of the digital divide (DeBell,
2003). A summary of these social and geo-political
effects is included in the Computer and Internet Use
by Students in 2003 report, published by the
National Center for Education Statistics in
September 2006, (DeBell, 2006). This report surveyed
about 56,000 households and obtained information on
close to 30,000 students from nursery school to 12th
grade. The results indicate that students’
technology usage is divided along low (87%) and high
(95%) income families; Whites (93%), Asian (91%),
Latinos (85%) and Blacks (86%); public (91%) or
private (86%) school enrollment; high-school (89%)
and college (93%) educated parents. All of these
differences are statistically significant at 0.05.
In anticipation of the less obvious future
challenges of education, and particularly the role
of IT-enhanced and multi-disciplinary curricula, we
need to debate and outline clear policies and
directions for smooth transitions and timely
resolutions of such unexpected conundrums. The ideas
proposed in this manuscript provide a starting point
for this debate on how to address prospectively two
of the most pressing needs of science and technology
education – the student-instructor Intergenerational
IT divide and the dilemma of effectively training
advanced discipline-bound and multidisciplinary
scientists.
&sp;
Acknowledgments
This research was supported in part by NSF DUE
grants 0442992 and 0716055, and by NIH Roadmap for
Medical Research, NCBC Grant U54 RR021813.
References
Adomavicius, G., Bockstedt, J., Gupta, A., &
Kauffman, R. J. (2006). Understanding Patterns of
Technology Evolution: An Ecosystem Perspective,
System Sciences, 2006. HICSS '06. Proceedings of the
39th Annual Hawaii International Conference on
(pp. 189a-189a).
Bender, D. M. (2005). Developing a collaborative
multidisciplinary online design course. The
Journal of Educators Online, 2,
http://www.thejeo.com/Diane%20Bender%20Final.pdf
Bongalos, Y. Q., Bulaon, D. D. R., Celedonio, L. P.,
de Guzman, A. B., & Ogarte, C. J. F. (2006).
University teachers' experiences in courseware
development. British Journal of Educational
Technology, 37, 695–704.
Borgman, C. L., Wallis, J.C., Enyedy, N. (2006).
Building digital libraries for scientific data: An
exploratory study of data practices in habitat
ecology., 10th European Conference on Digital
Libraries. Alicante, Spain: Springer.
Brescia, W., & Miller, M. (2006). What's it worth?
The perceived benefits of instructional blogging.
Electronic Journal for the Integration of Technology
in Education, 5, 44-52.
Burns, R. (1995). The Adult Learner at Work.
Sydney: Business and Professional.
Cartelli, A. (2006). Teaching in the knowledge
society: New skills and instruments for teachers.:
Hershey, PA: Idea Group Inc.
Conole, G., de Laat, M., Dillon, T., Darby, J.
(2008). `Disruptive technologies', `pedagogical
innovation': What's new Findings from an in-depth
study of students' use and perception of technology.
Computers & Education, 50, 511-524.
Cutrim, E. M., Rudge, D., Kits, K., Mitchell, J., &
Nogueira, R. (2006). Changing teaching techniques
and adapting new technologies to improve student
learning in an introductory meteorology and climate
course. Advances in Geosciences, 8,
11-18.
Daveri, F., & Maliranta, M. (2007). Age, seniority
and labour costs: lessons from the Finnish IT
revolution. Economic Policy, 22,
117–175.
DeBell, M. a. C., C (2006). Computer and Internet
Use by Students in 2003. Washington, DC: National
Center for Education Statistics. Available at:
http://nces.ed.gov/pubs2006/2006065.pdf
DeBell, M. a. C., C. (2003). Computer and Internet
use by Children and Adolescents in the United
States, 2001 (NCES 2004-014). Washington, DC:
U.S.: Department of Education, National Center for
Education Statistics.
Dinov, I. D., Boscardin, JW., Mega, MS., Sowell,
EL., Toga, AW. (2005). A Wavelet-Based Statistical
Analysis of fMRI data: I. Motivation and Data
Distribution Modeling. NeuroInformatics,
3, 319-343.
Dinov, I. D., Sanchez, J., & Christou, N. (2008).
Pedagogical Utilization and Assessment of the
Statistic Online Computational Resource in
Introductory Probability and Statistics Courses.
Journal of Computers & Education, 50,
284–300.
Finegold, D., Mohrman, S., & Spreitzer, G. (2002).
Age effects on the predictors of technical workers'
commitment and willingness to turnover. Journal
of Organizational Behavior, 23, 655-674.
Freeman, P. A., Crawford, D. L., Kim, S., Munoz, J.
L. A., Munoz J. L. (2005). Cyberinfrastructure for
Science and Engineering: Promises and Challenges.
Proceedings of the IEEE, 93, 682-691.
Guillot, L., & Pryor, S. (2007). PDA Use by
Undergraduate Nursing Students on Pediatric Clinical
Rotations. Journal of Hospital Librarianship,
7.
Hernon, P., & Schwartz, C. (2006). Recommendations
of the Commission on the Future of Higher Education.
Library & Information Science Research,
28, 477-479.
Holt, A., and Webb, T. (2007). Interdisciplinary
research: leading ecologists down the route to
sustainability? British Ecological Society,
38, 2-13.
Inoue, Y. E. (2006). Technology and Diversity in
Higher Education: New Challenges. Hershey, PA:
Information Science Publishing.
Joan, P., Peter, S., Jean-Yves, H., Ron, H., Miguel,
E. (2007). Increasing student retention in computer
science through research programs for
undergraduates, Proceedings of the 38th SIGCSE
technical symposium on Computer science education.
Covington, Kentucky, USA: ACM.
Koukel, S. D. (2006). Teaching styles and computer
use in family and consumer sciences teacher
education programs: a survey of university faculty
in Texas: Texas Tech University.
Maag, M. (2006). Podcasting and MP3 Players:
Emerging Education Technologies. Comput Inform
Nurs, 24, 9-13.
Mitrany, M., & Stokols, D. (2005). Gauging the
Transdisciplinary Qualities and Outcomes of Doctoral
Training Programs. Journal of Planning Education
and Research, 24, 437-449.
Moore, M. J. (2006). Adoption of new technologies in
instructional design [electronic resource] : a case
study of communications faculty at Florida Community
College at Jacksonville integrating an instructional
web-based writing tool, my ACCESS into
communications courses.: The University of Texas at
Austin. Available electronically from
http://hdl.handle.net/2152/420 .
Munakata, T. (2007). Introduction. Commun. ACM,
50, 30-34. DOI=
http://doi.acm.org/10.1145/1284621.1284643
Munshi, R., Coalson, R. D., Ermentrout, G. B.,
Mardura, J. D., Meirovitch, H., Stiles, J. R., and
Bahar, I. (2006). An introduction to stimulation and
visualization of biological systems at multiple
scales: a summer training program for
interdisciplinary research. Biotechnol. Prog.,
22, 179–185.
NAS
(2004). Committee on Facilitating Interdisciplinary
Research; National Academy of Sciences, National
Academy of Engineering, Institute of Medicine.
Facilitating interdisciplinary research. Washington:
The National Academies Press.
Norris, P. (2001). Digital Divide: Civic
Engagement, Information Poverty, and the Internet
Worldwide. New York: Cambridge University Press.
Pareek, D. (2006). WiMAX: Taking Wireless to the
MAX: Auerbach Publications.
Posch, A., & Steiner, G. (2006). Integrating
research and teaching on innovation for sustainable
development. International Journal of
Sustainability in Higher Education, 7,
276 - 292
Roberts, L. G. (2000). Beyond Moore's law:
Internet growth trends: IEEE Computer Mag.
Shekar, A. (2007). Active learning and reflection in
product development engineering education.
European Journal of Engineering Education,
32, 125–133.
Srivastava, A., Srivastava, A.,Joshi, S. H.,Mio,
W.,Xiuwen Liu, A. Xiuwen Liu (2005). Statistical
shape analysis: clustering, learning, and testing.
Pattern Analysis and Machine Intelligence, IEEE
Transactions on, 27, 590-602.
Vannatta, R. A., & Fordham, N. (2004). Teacher
dispositions as predictors of classroom technology
use. Journal of Research on Technology in
Education, 36, 253-271.
Willis, S., & Tranter, B. (2006). Beyond the
'digital divide': Internet diffusion and inequality
in Australia. Journal of Sociology, 42,
43-59.
Yaşar, O., Little, L., Tuzun, R., Rajasethupathy,
K., Maliekal, L., & Tahar, M. (2006).
Computational Math, Science, and Technology (CMST):
A Strategy to Improve STEM Workforce and Pedagogy to
Improve Math and Science Education: Springer
Berlin / Heidelberg
|