Introduction
Micro-level instructional design refers to the practice of designing and producing small units of instruction. Within the context of the online learning environment, micro-level design occurs during development of small multimedia products such as short presentations, podcasts, or interactive simulations with a duration of approximately one to fifteen minutes. The content included within each micro-product may be selected based on the idea of chunking, which involves organizing conceptually related information into units. The size of a chunk of content increases with the complexity of the information (Hannafin & Hooper, 1993). Micro-multimedia can be played on computers or it can be downloaded onto mobile media devices to be carried to remote locations for field-based work. This supports new possibilities for online instruction. However, there is an apparent lack of a comprehensive framework to guide micro-level design (Koumi, 2003; Sedig & Rowhani, 2005). Traditional models of instructional design are geared toward development of larger units of instruction (Dick, Carey, & Carey, 2005; Peterson, 2003). Because of this, the scope goes beyond what is needed for micro-design. At the micro-level processes such as shifting focus to small-scale design, applying learning theory, managing the technology, and evaluating the micro-design are engaged. This paper illustrates how these processes can be conceptualized within the context of a multimedia-enhanced online course.
Shifting Focus to Small-Scale Design
Online course design is a complex process involving planning and development spanning multiple levels of scale. The focus of attention during design can shift in magnification from broad institutional goals, down to course-level curricular decisions, and even further down to the level of instructional practice. This is the micro level where the products of education are designed and constructed. With respect to content development this includes products such as multimedia presentations, video clips, or audio podcasts that a course designer selects or creates for inclusion in an online course. The terms micro, meso, and macro have been used previously to describe both instructional products and instructional design. There are three perspectives that may be considered when designing instructional technology products. ”The micro-level perspective refers to a technology product in itself, the meso-level to the product as it is part of a usage situation, and the macro level to the product in terms of its implications in a larger context“ (Collis, 2002, p. 2). The macro, meso, and micro perspectives correspond to decreasing levels of granularity for the design of educational products such as multimedia-enhanced online courses. At the macro-level decisions about the design of an online course are situated within a larger program or institution. From this higher level of granularity course design is geared toward alignment of curricular goals with programmatic and institutional needs. The meso-level shifts to a narrower focus of design granularity as the overarching framework of a course is mapped out for a specific context. The micro-level of design assumes an even finer focus toward development of individual elements of instruction.
The term micro-level design has been in existence for at least twenty years. Reigeluth and Curtis (1987) used the term when describing the process whereby each individual skill or piece of information within an instructional sequence is designed. Micro-level design also includes micro-level strategies that consist of components such as “…examples, practice, feedback, representation forms, memory devices, and attention-focusing devices” (Reigeluth & Curtis, 1987, p. 190). These components are easily integrated into an online course through micro-level structures such as multimedia learning objects and instructional messages. A learning object is a type of micro-structure that has received considerable attention in recent years. The precise definition of the term “learning object” remains illusive. However, Moisey and Ally (2007) argue that three characteristics commonly associated with learning objects are that “…they are digital, they support learning, and they are reusable…” (p. 324). Reusability is supported through content management standards such as SCORM (Sharable Content Object Reference Model) from the Advanced Distributed Learning Project (http://www.adlnet.gov/). SCORM supports packaging, delivery, and usage tracking of e-learning content in learning management systems (Carnegie Mellon Learning Systems Architecture Lab, 2004).
If learning objects are reusable as units of instructional content then it is possible for them to be shared among multiple learning contexts. By contrast, an instructional message is a micro-structure that may or may not stand on its own. The focus is on communication and presentation of information. According to Mayer (2001), “An instructional message is a communication that is intended to foster learning” (p. 3). When words and pictures are combined to form a presentation then a multimedia instructional message is created. In an online course, instructional messages are fundamental communication units subsumed within other structures including learning objects and other forms of instructional content. Within a single course there could be hundreds of instructional messages serving multiple purposes.
In online classrooms instructional messages are composed of digital representations including text, images, video, audio, and animations. Representations are the essential building blocks of micro-level design. Digital representations enable creation of asynchronous instructional messages, which store information in a permanent form for anytime access. Although online instruction can occur in various forms including hybrid and Web-enhanced courses, Allen and Seaman (2006) have defined an online course as one “…in which at least 80 percent of the course content is delivered online” (p. 4). This definition suggests a critical role for the information presented to students on a course Website. One obvious reason for this is that students typically participate in online courses at various times from diverse locations. It is important to have high quality course information and content waiting for them when they visit the site. Since they are more likely to be on their own, it is critical that the course content is well designed so that confusion and frustration is minimized.
One of the benefits of the electronic classroom lies in the ability to integrate multimedia representations that take advantage of a broad set of communicative properties (Snelson, 2005). For example, an online instructor might wish to explain the Doppler Effect using the example of a train whistle that changes pitch as the train passes by. This can be handled through text alone, but the instructional message can communicate the idea more effective by integrating audio and animation. Through audio students can hear the Doppler Effect in the passing train whistle. The addition of an animated diagram that is synchronized with the sound can show what is happening with the sound waves during the pitch change. In this way students simultaneously see the diagram while listening to the corresponding sound pitch change during the Doppler Effect. A screenshot showing an example of an online movie depicting the Doppler Effect is shown in Figure 1. The working example is available online at: http://edtech.boisestate.edu/snelsonc/examples/doppler.html .
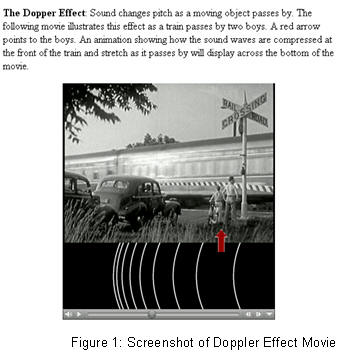
The example of the Doppler Effect instructional message demonstrates how multiple representations can be clustered to utilize the benefits of their combined properties. The term computational efficiency (Larkin & Simon, 1987) has been used to describe the ease with which information can be extracted from the representations. For example, spatial information may be easier to read from a diagram than from a text description. The diagram in Figure 2 illustrates this concept. The spatial information is easier to read from the diagram of the table with names surrounding it than it is from the text description.
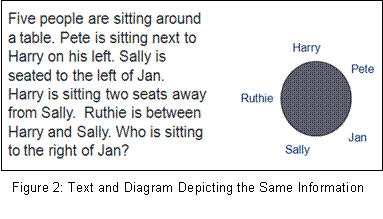
When groups of multiple representations are combined they form a system of representations with each contributing its set of computational properties. In addition to this, each representation functions within the system to complement, constrain, or construct (Ainsworth, 1999). Representations complement when presenting information in more than one form such as when a text description is presented with a corresponding image depicting the same idea. A text description of a cumulous cloud is complemented when a photo showing a cumulous cloud is also provided as shown in Figure 3.
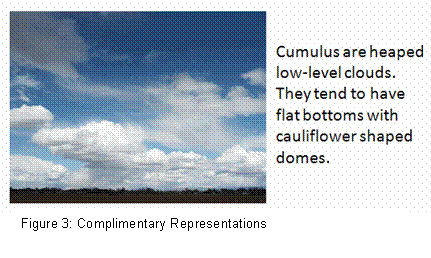
The constraining function occurs when a representation helps to reduce misunderstanding. Text directions for folding a paper airplane can state that one edge of the paper needs to be folded toward the center, but it may be unclear which edge to fold. A diagram placed with the text can function to constrain misunderstanding by illustrating where the fold should be made as illustrated in Figure 4.
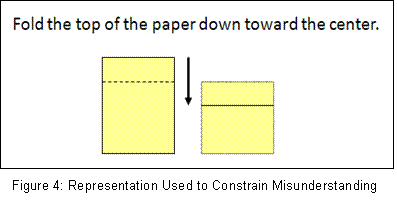
The constructing function of representation is used to elicit greater levels of abstraction or generality. To see how this works, consider an example where the definition of a quadrilateral as a four sided figure is described in text. If an image of a square is provided, it shows one instance of a quadrilateral, but may not lead the learner to understand the more general idea of quadrilaterals. However, if a whole group of four sided shapes are shown in an image within the representational system a greater level of generality with respect to the bigger idea of quadrilaterals is communicated as illustrated in Figure 5.
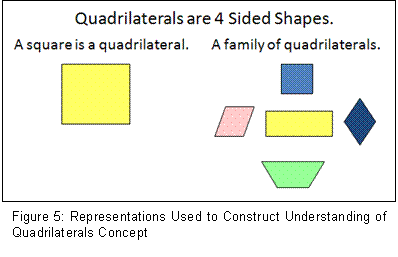
The three functions of representation can be used in micro-level design to craft instructional messages with multiple forms of representation. This extends to multimedia instructional message design where the properties of dynamic representations may also be incorporated in the system. The communicative aspects of representations are beneficial in micro-level design, particularly when combined with additional principles derived from psychology and learning theory.
Applying Learning Theory
The potential for micro-multimedia to positively affect learning can be increased when applying principles from learning theory. According to Mayer (2001) “Multimedia messages that are designed in light of how the human mind works are more likely to lead to meaningful learning than those that are not” (p. 41). The cognitive theory of multimedia learning describes how information in words and pictures is processed by learners in two separate channels beginning with the eyes and ears (Mayer, 2005). Within working memory visual and verbal information in the two channels is combined, organized, and integrated with prior learning previously stored in long term memory. Several research-based principles of multimedia learning that align with this model have been derived from multiple studies (Mayer, 2001). Some of these principles relate to the format, selection, or arrangement of the representations in the multimedia message. For example, the combination of words and pictures is preferable to words alone for promoting higher levels of student learning with respect to retention and transfer of knowledge (multimedia principle). It is preferable to position corresponding words and pictures near each other (spatial contiguity) and present them simultaneously (temporal contiguity). In animated presentations it is preferable to include audio narration (modality principle) and avoid the addition of redundant on screen text (redundancy principle). Extraneous information such as background sound is interesting, but marginally applicable information (i.e. seductive details) or media such as unnecessary background sound is best left out of multimedia messages (coherence principle).
One of the underlying assumptions of the cognitive theory of multimedia learning is that humans have limited information processing capabilities in working memory (Mayer, 2005). When this capacity is exceeded, the subsequent cognitive load interferes with learning. Cognitive load is often introduced through poor instructional design where limits on working memory are ignored (Sweller, 2005). A split-attention effect occurs when the learner is forced to divide attention between multiple sources of information within an instructional message. An example of this is when two or more forms of visual information compete for attention. An animated diagram containing blocks of on screen text forces attention to be divided between two visual representations. The diagram and text both require the visual channel for entry into the learner’s sensory system. In situations like this cognitive load can be reduced by applying the modality and temporal contiguity principles of multimedia learning (Mayer & Moreno, 2003). To accomplish this, the text needs to be recorded as an audio narration, which is then synchronized with the animated diagram. Visual and auditory channels can work together to process the information and reduce cognitive load.
Cognitive load is increased when visual and auditory channels are overloaded with more than they can process. However, representational redundancy is not the only thing that can contribute to cognitive load problems. Task complexity is another factor that may cause cognitive overload. In recent years, it has become popular to provide minimal guidance to learners while immersing them in discovery, inquiry, problem-based, and experiential forms of instruction. The appeal of minimally guided instruction appears to center in the promise of rich learning experiences encountered when engaging in authentic problems and processes. This approach seems engaging and may be valuable in some cases. However, minimally guided instruction has been called into question as having little evidence to support its effectiveness (Kirschner, Sweller, & Clark, 2006).
Problems with minimally guided approaches to instruction are related to incompatibility with human cognitive architecture, expert-novice differences, and cognitive overload. For novice learners, cognitive load is increased when immersing them in situations that require at least some prior knowledge. An example would be the situation of a student who has been asked to create a Web page and post it on the Internet. If this student has no experience with the task and is given no guidance, the student will spend a lot of time trying to understand how to do the assignment. Without prior exposure to technical terminology or processes involved in this task students may not even know how to begin. Some direct instruction can assist students with the process of developing an essential mental model, or schema, of Web page authoring. This approach for the novice learner has the opportunity to reduce cognitive load on working memory.
Direct instruction is defined as a teaching method characterized by a combination of instructor presentation and student practice (Joyce, Weil, & Calhoun, 2000). It is not simply a lecture although there is direct explanation of concepts and procedures. At the present time direct instruction is not always considered the optimal choice. Magliaro, Lockee, and Burton (2005) explained that direct instruction “…has become the whipping post in some pedagogical camps, while the panacea in others” (p. 41). At the micro-level of instructional design direct teaching is done in very small amounts. Small units of instruction can be used to minimize cognitive load by providing strategically placed just-in-time information within complex learning situations.
Micro-level multimedia may also be used to reduce cognitive load through scaffolding, which is the practice of providing support for learners and then gradually reducing that support (van Merrienboer, Kirschner, & Kester, 2003). Small multimedia presentations, when strategically placed within an online course, can help learners get started with complex tasks. Scaffolding is a strategy that has been associated with constructivist learning environments (Jonassen, 1999). Constructivism refers to the manner in which learners construct meaning based on interpretation of their experiences both individually and socially. It has been associated with minimally guided instruction such as discovery learning. However, Mayer (1999) has argued that “…learners can construct meaning from well-designed direct instruction…” (p. 143). From this perspective constructivism is dependent on the learner’s mental engagement and cognitive activity.
In online instruction, students have considerable control over the level of engagement they choose to pursue when using course materials. Multimedia can be attached to a course site through hyperlinks enabling more advanced students to ignore information that they already comprehend. Novices can use the basic information while the more expert learners can pass it by providing a type of differentiation through self-selection. The drawback is that students may bypass instructio actually need due to overestimation of their abilities, lack of interest, or time constraints. Online courses require good self-regulation by students because they are responsible to login, read course materials, view multimedia presentations, and complete assignments. Self-regulation has been associated with aspects of learner volition such as motivation and action control (Deimann & Keller, 2006). It seems logical to conclude that motivation is an important contributing factor behind student participation and success in an online course. In fact, an integrated model of multimedia learning has been proposed by Astleitner and Wiesner (2004) who extended the cognitive theory of multimedia learning (Mayer, 2005) by adding a motivation component.
A positive relationship between motivation and multimedia seems probable. However, Clark and Feldon (2005) have argued that “…evidence for the motivational qualities of multimedia instruction has been elusive at best” (p. 101). Simply putting multimedia in a course does not guarantee enhanced motivation, nor does it mean motivation will fail to occur. However, motivation principles of instructional message design suggest that variation and curiosity can be used to help gain learner attention (Keller & Burkman, 1993). Online courses can be augmented with small multimedia presentations to break up the monotony of long text passages and take advantage of the communicative properties of multiple representations. Curiosity is a powerful motivator. By varying the instructional materials it is possible to invoke curiosity and interest. Park and Lim (2007) conducted an experiment to examine the effect of visual illustrations on interest, achievement, and motivation as compared to plain text. While no effect was found for achievement, a positive effect was found for interest and motivation variables. Park and Lim wrote that “The findings of the study suggest that using illustration in multimedia instructional material increases learners’ learning interest and motivation” (p. 159).
The first event of instruction, according to Gagné and Medsker (1996) is to gain learner attention. Multimedia presentations within an online course can be effectively used to gain attention, especially if they introduce the elements of variation and curiosity. Attention is also the first component of the well known ARCS model of motivation, which has been validated through research conducted in the context of multinational e-learning environments (Keller, 1999; Keller & Suzuki, 2004). The ARCS acronym stands for Attention, Relevance, Confidence, and Satisfaction. Learner attention is gained through “…the use of interesting graphics, animation or any kind of event that introduces incongruity or conflict” (Keller & Suzuki, 2004, p. 231). Variability is an important factor for gaining and maintaining attention. At the micro-design level this implies that variation of the multimedia elements in an online course may stimulate student interest.
Another possible motivational feature that could be introduced with multimedia is the human factor. Students are separated from each other and the instructor in an asynchronous online course. All communication is mediated through digital representation. Short video and audio clips enable members of the class to see and hear each other. This motivational feature could make the course seem less sterile as human faces and voices are integrated. This idea is supported by findings from a study conducted to explore student reactions to small multimedia presentations integrated into online courses. Student comments on anonymous evaluations of micro-level multimedia consistently reported an appreciation for the presence of a human voice in the presentations (Snelson, 2007). This relates to the idea of teacher immediacy, which refers to the physical or psychological closeness perceived between communicators (Allen, Witt, & Wheeless, 2006).
It is apparent that application of instructional theory to micro-level design involves consideration of multiple related principles. While the design of multimedia for learning in an online learning environment is improved when applying these principles this is only a part of micro-level multimedia design. There are additional technical factors to complement the design in order to make the multimedia functional in the online classroom.
Managing the Technology
One of the first technical factors to manage with Web-based multimedia is file size. Broadband access has increased; however, it remains varied and unequal. A data memo published on the Pew Internet & American Life Project Website reported survey results from 2,200 Americans that was conducted in early 2007 to measure home broadband penetration in the United States (Horrigan & Smith, 2007). Results from this survey revealed that only 47% of the respondents had high-speed Internet connections in their homes and 15% were using slower dial-up access. The remaining respondents accessed the Internet from work or other locations, did not use the Internet at all, or failed to specify a connection type. Even when high-speed broadband Internet access is available in U.S. homes or businesses the data transfer rates falls below other countries. The speed with which files may be downloaded affects what is possible on the Internet, thus affecting feasibility of multimedia integration in online courses. Large multimedia files can become unwieldy for students who are using slower Internet connections. For example, something as small as a four minute video with a file size of 3 megabytes might take 30 seconds to download through high-speed broadband access, yet take a cumbersome 10 minutes or longer to download on slower connections such as 56 Kbps (kilobyte per second) dial up. The issue is further compounded by global differences in broadband download speed capacity. A report published by the Communications Workers of America (2007) indicates that Internet download speeds in the U.S. fall below other countries including Canada, France, Sweden, Finland, South Korea, and Japan. The difference is greatest between the U.S. and Japan, which has a median download speed 30 times faster than the U.S.
Because high speed access and capacity is so varied it is necessary to design multimedia with minimal file sizes. One strategy is to apply compression techniques to reduce sizes. The application of a codec (compressor/decompressor) to multimedia can drastically reduce the size of the file and make it more manageable for Internet use. For example, a 25 second video clip that is saved in uncompressed AVI format can be reduced from 88 megabytes down to 1.8 megabytes by compressing it into Flash® video. The resulting file size is about 2% of the original. The process of compression is essential for multimedia that is to be viewed or downloaded from the Internet. Another way to shrink a multimedia presentation is to reduce the height and width dimensions. The 25 second 88 megabyte AVI video clip with dimensions of 640 pixels by 480 pixels can be shrunk to just under 7 megabytes by reducing the dimensions to half size. The main drawback to this approach is that the viewing quality might be reduced as well. Sometimes it is difficult to see what is being shown in a video with tiny screen size.
Compression and reduction of the height and width dimensions are two ways to reduce file size. Another method is to restrict the duration of multimedia files. Shorter presentations contain less content and lower file size. When the 25 second video clip is extended to one minute the file bloats from 88 megabytes to 214 megabytes. Even after compression longer videos or audio presentations reach a point where they become inaccessible for students who are attending the online class via dial-up access. It is essential to keep each video clip as short as possible while maintaining sufficient content to make it useful. Longer clips and presentations can be broken down into a series of presentations if necessary. While these technical suggestions may not appeal to all people who design or teach online, it is nevertheless important to consider feasibility issues when delivering multimedia content across the Internet.
Micro-level design of short and small sized bits of media is suitable for micro-media devices such as cell phones, iPods, PDAs (portable digital assistants) and other hand-held mobile devices. The storage and multimedia capabilities of these devices has evolved to a point where it is now possible to download video, audio, or Web page content. Strategies for integrating these technologies have found their way into higher education. Universities such as Stanford (http://itunes.stanford.edu/) and UC Berkeley (http://itunes.berkeley.edu/) have established iTunes U Websites where students may download lectures, interviews, music, sports, and more (http://www.apple.com/education/itunesu/). These digital multimedia files may be loaded onto an iPod to be carried around with the user.
At first glance, it seems like an exciting new world for education with this proliferation of high-powered micro-media and the promise of new possibilities for teaching and learning. Yet, the rush to embrace new and trendy technology without a clear understanding of best instructional practice may be ill advised. This potential problem is reminiscent of issues raised throughout the long standing and unresolved media-effects debate. In this debate, Clark (1994) has argued that instructional method rather than media is the primary force affecting learning. This argument is based on the premise that similar instructional methods can be presented in more than one medium. Meta analysis studies of distance education research literature support this point of view (Bernard et al., 2004; Lou, Bernard, & Abrami, 2006).
An alternative viewpoint in the media effects debate is that the attributes of different types of media enable unique capabilities such as dynamic visualization and interactivity (Kozma, 1994). The new micro-media has the attribute of portability, which supports learner mobility. Multimedia can be transported to remote locations, thus expanding possibilities for field work within online instruction. Since mobile devices plug into the computer the possibility also exists for students to upload video, audio, or data recordings to submit as part of an assignment within an online course. Testing and evaluation of micro-level instructional design is critical in determining how and to what extent these new teaching strategies and micro-media devices support teaching and learning outcomes.
Evaluating the Micro- Design
Although micro-level instructional design has appeared in various forms, it has been poorly defined as a design field. Principles for micro-level design may be extracted from the literature, but a coherent structure is elusive. Koumi (2003) wrote “…there appears to be no published comprehensive framework of micro-level design principles for optimal integration of visuals and audio” (p. 19). A call has been made to increase micro-level multimedia learning research to help build such a framework. This has been referred to as a new generation of multimedia learning research, which ideally “…should lead to a comprehensive framework of principles at the micro-level for the design of effective multimedia learning systems” (Samaras, Giouvanakis, Bousiou, & Tarabanis, 2006, p. 20). Implied here is the underlying idea of practical relevance. This relates directly to the question of how (or even if) research findings will manifest into principles that designers can use in the real world.
Concerns about the divide between educational research findings and everyday practice have contributed to the emergence of design-based research (DBR). DBR is “…an emerging paradigm for the study of learning in context through the systematic design and study of instructional strategies and tools” (The Design Based Research Collective, 2003, p. 5). It is characterized by longitudinal cycles of design, implementation, analysis, and redesign within a real-world learning context. The cycles of design-based research have been described as comprising of short duration microcycles within longer macrocycles (Gravemeijer & Cobb, 2006; Jonassen, Cernusca, & Ionas, 2007). The microcycles loop within the longer macrocycles. Within an online course the duration of a microcycle can be defined as a weekly assignment phase or as the implementation event for each micro-level multimedia product. Each piece of multimedia can then be evaluated within the longer course cycle, which constitutes the macrocycle. During a macrocyle, the same group of learners progress through the course using the micro-level multimedia designs within a common context. As each micro-level multimedia product is implemented and tested within the naturalistic learning environment, adjustments can be made to the design and findings are related back to the guiding learning theory. Subsequent macrocycles with different groups of learners or in different contexts serve as comparison groups.
DBR has been described as valuable for studies conducted in technology-enhanced learning environments (Wang & Hannafin, 2005). A virtual form of DBR, called virtual design based research (Snelson, 2006), has been used to evaluate micro-level multimedia designs in online instruction (Snelson, 2007). In this research, an anonymous tracking system was designed to explore usage levels of micro-level multimedia instructional messages. Anonymous and voluntary student evaluations, tracked by a course identification number, were collected for each implementation of the multimedia messages. This system provided a way to obtain candid feedback from students without rousing concerns about coercion or retaliation. This provided some feedback, to aid in the formative evaluation process. However, it should be noted that the sample was self-selected and may not be representative of the entire group of learners. Technology played a vital role for virtual observation and automatic data collection. During each microcycle, new multimedia instructional messages were released and data was collected for immediate analysis. Design revisions based on insights gained during analysis of the data were implemented in subsequent microcycles. Comparisons were made from one microcycle to the next. Additional comparisons were made from one course to another to compare results between implementations with different groups of students. This was possible because principles of multimedia learning and instructional message design were applied when designing all of the micro-multimedia. By continually relating design decisions to theory and evaluation data, DBR can shift to a level of generality above action research or formative evaluation with respect to applicability beyond the local context.
Kelly (2004) has suggested that design studies contribute to the process of hypothesis and framework generation. This may be one of the essential roles DBR plays within the spectrum of research methodologies. The cycles of design, implementation, and analysis generate new questions, hypotheses, and design principles that may contribute to the development and validation of a framework of micro-level instructional design. This not only supports immediate evaluation goals within a local context, but may suggest possible theories of micro-level design for further study within multiple contexts.
DBR could also be coordinated with more conventional forms of research to explore design variables from multiple perspectives. For instance, insights into how the mind works can be studied in experimental laboratories. Results from such experiments may generate new theoretical conjectures about learning with multimedia. New micro-level multimedia products could then be designed to test the conjectures within real-world classrooms. Implementation would likely yield new hypotheses and additional conjectures for further study. However, one of the challenges in coordinating research between DBR and the laboratory is finding conjectures which lend themselves well to meaningful investigation in both environments. Collaboration in this situation is facilitated by developing operational definitions of outcomes that specify clear agreement of the meaning of variables under study (McCandliss, Kalchman, & Bryant, 2003). Although potentially difficult, this exchange could produce profound knowledge to inform the practice of micro-level instructional design of multimedia for online instruction.
Conclusions and Implications
Micro-level design is gaining momentum as an emerging design field. An Internet search reveals that terms such as micro-learning and nano-learning appear on multiple Websites (http://www.microlearning.org/ and http://www.clomedia.com/content/templates/clo_article.asp?articleid=1221). Although largely driven by the evolution of new mobile media devices, micro-level design is essential when designing multimedia for delivery via the Internet. When scaling the design focus down to the micro level, online course designers can apply principles from learning theory to craft effective multimedia messages. By considering technical factors associated with the virtual learning environment, multimedia may be produced in a form that is accessible to students who have variable types of Internet access. Evaluation, which is a characteristic of all instructional design models, may be conducted through iterative cycles using a virtual design based research approach. The Web server technology necessary for course delivery can be used to collect, store, and process data. When coordinated with other forms of research, such as controlled laboratory studies, new theories of design for micro-multimedia may be derived and used to build a cohesive framework to guide practice. Future work in this area is needed to produce operational definitions and devise research protocols to coordinate these efforts. Additional research is needed to produce examples of micro-level multimedia that may serve as prototypes of general design principles. Through this process, a research-based framework of micro-level instructional design can emerge to guide designer of multimedia for online instruction.
References
Ainsworth, S. (1999). The functions of multiple representations. Computers & Education, 33(2/3), 131-152.
Allen, I. E., & Seaman, J. (2006). Making the Grade: Online education in the United States, 2006. Neeham and Wellesley, MA: The Sloan Consortium. Retrieved August 20, 2007 from http://www.sloan-c.org/publications/survey/index.asp
Allen, M., Witt, P. L., & Wheeless, L. P. (2006). The role of teacher immediacy as a motivational factor in student learning: Using meta-analysis to test a causal model. Communication Education, 55(1), 21-31.
Astleitner, H., & Wiesner, C. (2004). An integrated model of multimedia learning and motivation. Journal of Educational Multimedia and Hypermedia, 13(1), 3-21.
Bernard, R. M., Abrami, P. C., Lou, Y., Borokhovski, E., Wade, A., Wozney, L., et al. (2004). How does distance education compare with classroom instruction? A meta-analysis of the empirical literature. Review of Educational Research, 74(3), 379-439.
Carnegie Mellon Learning Systems Architecture Lab. (2004). SCORM best practices guide for content developers. Retrieved November 10, 2007, from http://www.jointadlcolab.org/downloads/scormcdg_1ed_sec7update_20050420.pdf?
Clark, R. E. (1994). Media will never influence learning. Educational Technology Research & Development, 42(2), 21-29.
Clark, R. E., & Feldon, D. E. (2005). Five common but questionable principles of multimedia learning. In R. E. Mayer (Ed.), The cambridge handbook of multimedia learning (pp. 97-115). New York: Cambridge University Press.
Collis, B. (2002). Information technologies for education and training. In H. H. Adelsberger, B. Collis & J. M. Pawlowski (Eds.), Handbook on information technologies for education and training (pp. 1-22). New York: Springer.
Communication Workers of America (2007). Speed Matters: A report on Internet speeds in all 50 states. Retrieved August 20, 2007, from http://www.speedmatters.org/document-library/sourcematerials/sm_report.pdf.
Deimann, M., & Keller, J. M. (2006). Volitional aspects of multimedia learning. Journal of Educational Multimedia and Hypermedia, 15(2), 137-158.
Dick, W., Carey, L., & Carey, J. (2005). The systematic design of instruction (6th ed.). New York: Pearson.
Gagne', R. M., & Medsker, K. L. (1996). The conditions of learning: Training applications. Fort Worth, Texas: Harcourt Brace College Publishers.
Gravemeijer, K., & Cobb, P. (2006). Design research from a learning design perspective. In J. Van Den Akker, K. Gravemeijer, S. McKenney & N. Nieveen (Eds.), Educational design research (pp. 17-51). London: Routledge.
Hannafin, M. J., & Hooper, S. R. (1993). Learning principles. In M. Fleming & W. H. Levie (Eds.), Instructional message design: Principles from the behavioral and cognitive sciences (2nd ed.). Englewood Cliffs, NJ: Educational Technology Publications.
Horrigan, J. B., & Smith, A. (2007). Home broadband adoption 2007. Retrieved August 20, 2007, from http://www.pewinternet.org/pdfs/PIP_Broadband%202007.pdf
Jonassen, D. (1999). Designing constructivist learning environments. In C. M. Reigeluth (Ed.), Instructional-design theories and models: A new paradigm of instructional theory (Vol. 2, pp. 215-239). Mahwah, NJ: Lawrence Erlbaum Associates, Inc.
Jonassen, D., Cernusca, D., & Ionas, G. (2007). Constructivism and instructional design: The emergence of the learning sciences and design research. In R. A. Reiser & J. V. Dempsey (Eds.), Trends and issues in instructional design and technology (2nd ed., pp. 45-52). Upper Saddle River, NJ: Pearson.
Joyce, B., Weil, M., & Calhoun, E. (2000). Models of teaching (6th ed.). Boston: Allyn and Bacon.
Keller, J. M. (1999). Using the ARCS motivational process in computer-based instruction and distance education. New Directions for Teaching & Learning(78), 39-47.
Keller, J. M., & Burkman, E. (1993). Motivation Principles. In M. Fleming & W. H. Levie (Eds.), Instructional message design: Principles from the behaviorial and cognitive sciences (pp. 3-53). Englewood Cliffs, NJ: Educational Technology Publications.
Keller, J. M., & Suzuki, K. (2004). Learner motivation and e-learning design: A multinationally validated process. Journal of Educational Media, 29(3), 229-239.
Kelly, A. E. (2004). Design research in education: Yes, but is it methodological? . Journal of the Learning Sciences, 13(1), 115-128.
Kirschner, P. A., Sweller, J., & Clark, R. E. (2006). Why minimal guidance during instruction does not work: An analysis of the failure of constructivist, discovery, problem-based, experiential, and inquiry-based teaching. Educational Psychologist, 41(2), 75-86.
Koumi, J. (2003). Synergy between audio commentary and visuals in multimedia packages. Learning, Media and Technology, 28(1), 19-34.
Kozma, R. B. (1994). Will media influence learning? Reframing the debate. Educational Technology Research & Development, 42(2), 7-19.
Larkin, J. H., & Simon, H. A. (1987). Why a diagram is (sometimes) worth ten thousand words. Cognitive Science, 11(1), 65-99.
Lou, Y., Bernard, R. M., & Abrami, P. C. (2006). Media and pedagogy in undergraduate distance education: A theory-based meta-analysis of empirical literature. Educational Technology Research and Development 54(2), 141-176.
Magliaro, S. G., Lockee, B. B., & Burton, J. K. (2005). Direct instruction revisited: A key model for instructional technology. Educational Technology Research & Development, 53(4), 41-55.
Mayer, R. E. (1999). Designing instruction for constructivist learning. In C. M. Reigeluth (Ed.), Instructional-design theories and models: A new paradigm of instructional theory (Vol. 2, pp. 141-159). Mahwah, NJ: Lawrence Erlbaum Associates, Inc.
Mayer, R. E. (2001). Multimedia learning. New York: Cambridge University Press.
Mayer, R. E. (2005). Cognitive theory of multimedia learning. In R. E. Mayer (Ed.), The cambridge handbook of multimedia learning (pp. 31-48). New York: Cambridge University Press.
Mayer, R. E., & Moreno, R. (2003). Nine ways to reduce cognitive load in multimedia learning. Educational Psychologist, 38(1), 43-52.
McCandliss, B. D., Kalchman, M., & Bryant, P. (2003). Design experiments and laboratory approaches to learning: Steps toward collaborative exchange. Educational Researcher, 32(1), 14-16.
Moisey, S., & Ally, M. (2007). Fulfilling the promise of learning objects. In M. G. Moore (Ed.), Handbook of distance education (pp. 323-337). Mahwah, NJ: Lawrence Erlbaum Associates.
Park, S., & Lim, J. (2007). Promoting positive emotion in multimedia learning using visual illustrations. Journal of Educational Multimedia and Hypermedia, 16(2), 141-162.
Peterson, C. (2003). Bringing ADDIE to life: Instructional design at Its best. Journal of Educational Multimedia and Hypermedia, 12(3), 227-241.
Reigeluth, C. M., & Curtis, R. V. (1987). Learning situations and instructional models. In R. M. Gagne (Ed.), Instructonal technology foundations (pp. 175-206). Mahwah, NJ: Lawrence Erlbaum Associates.
Samaras, H., Giouvanakis, T., Bousiou, D., & Tarabanis, K. (2006). Towards a new generation of multimedia learning research. AACE Journal, 14(1), 3-30.
Sedig, K., & Rowhani, S. (2005). Micro-level design of interactive visual learning environments. In Proceedings of World Conference on Educational Multimedia, Hypermedia and Telecommunications 2005 (pp. 1050-1057). Chesapeake, VA: AACE.
Snelson, C. (2005). Designing dynamic online lessons with multimedia representations. The Journal of Educators Online, 2(1), Retrieved August 20, 2007, from http://www.thejeo.com/Archives/Volume2Number1/V2N1.htm.
Snelson, C. (2006). Virtual design based research. Academic Exchange Quarterly, 10(4), 107-110.
Snelson, C. (2007). Virtual design based research for multimedia integration studies. Proceedings of the World Conference on Educational Multimedia, Hypermedia & Telecommunications, Chesapeake, VA: AACE, 2007(1), 621-630.
Sweller, J. (2005). Implications of cognitive load theory for multimedia learning. In R. E. Mayer (Ed.), The cambridge handbook of multimedia learning (pp. 19-30). New York: Cambridge University Press.
The Design Based Research Collective (2003). Design-based research: An emerging paradigm for educational inquiry. Educational Researcher, 32(1), 5-8.
van Merrienboer, J. J. G., Kirschner, P. A., & Kester, L. (2003). Taking the load off a learner's mind: Instructional design for complex learning. Educational Psychologist, 38(1), 5-13.
Wang, F., & Hannafin, M. J. (2005). Design-based research and technology-enhanced learning environments. Educational Technology Research & Development, 53(4), 5-23.
|